Antimatter atoms trapped for 16 minutes
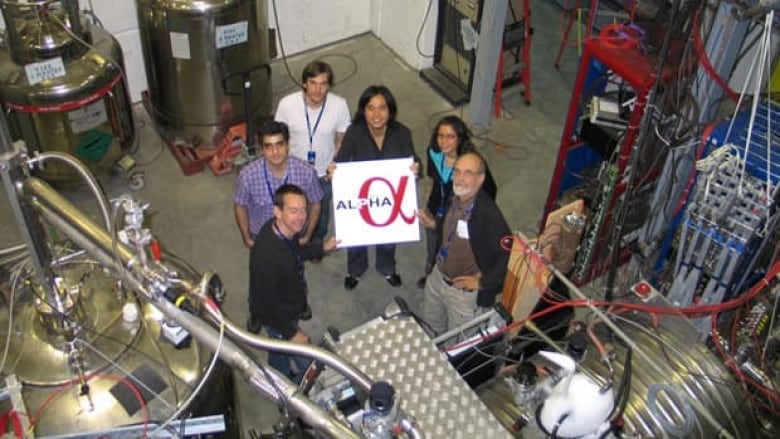
Antimatter atoms have been held captive and kept in existence for a whopping 16 minutes by a Canadian-led team — far longer than the researchers thought possible.
"It was quite a surprise," said Makoto Fujiwara, lead author of a study published Sunday in Nature Physics. It reported trapping antiatoms of antihydrogen — the antimatter counterpart of a hydrogen atom — for 1,000 seconds.
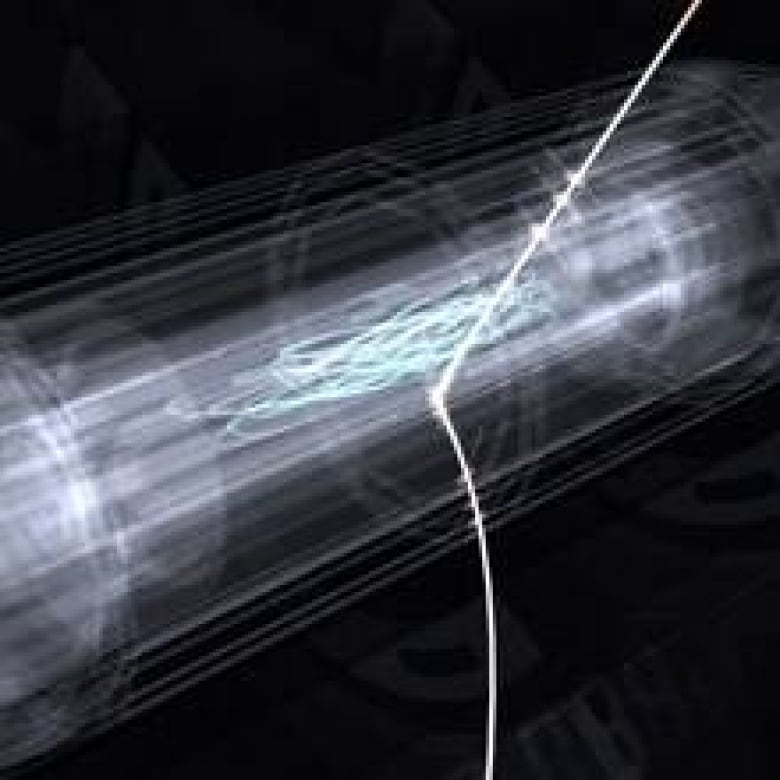
Antimatter is very difficult to keep in existence because the moment it touches matter, which makes up most of our universe, both the matter and antimatter are annihilated, producing pure energy.
The scientists' recent achievement has extended the experimental lifetime of antihydrogen atoms 5,000-fold since the ALPHA experiment — an international collaboration Fujiwara is part of — first figured out how to trap them at all.
The team, based at the laboratory of CERN, the European organization for nuclear research, near Geneva, published its method in Nature last November. At the time, it reported that it had held onto the antiatoms for less than one-fifth of a second.
Holding antiatoms captive for several minutes opens up a new range of possible experiments to probe the nature of antimatter, said Fujiwara, a research scientist at Vancouver-based TRIUMF and an adjunct professor at the University of Calgary.
'Game changer'
Scientists will more easily be able to do experiments to compare antihydrogen to hydrogen and could even potentially test how antimatter atoms are affected by gravity, he added. "I call it a game changer."
The trouble with antimatter
Antimatter is produced in equal quantities with matter when energy is converted into mass — this happens in particle colliders and is believed to have happened during the Big Bang at the beginning of the universe. That's why physicists are puzzled about why there is no longer a significant amount of antimatter in the universe.
But it's very difficult to study antimatter in order to answer such questions because antimatter and matter are both annihilated the moment they encounter each other, producing pure energy.
The problem is our world is made almost entirely of matter, including the walls of any container that might be used to hold antimatter.
Charged antiparticles can be kept away from the walls of a matter-based container using electric fields, but neutral antiatoms are much harder to trap, and are typically annihilated as soon as they are created.
Last November, the ALPHA team reported that it managed to trap antiatoms by taking advantage of a very tiny, weak magnet inside each atom. They created the antiatoms by mixing positrons and antiprotons very carefully inside a "magnetic trap" — a magnetic field generated by a powerful superconducting magnet. If the newly formed antiatoms were extremely cold and moving very slowly, the extremely weak magnetic force was enough to keep them from hitting the walls of the container.
After the experiment, the antiatoms are let out of the trap and they collide with matter, producing an energy signal that confirms that antimatter had been inside the container.
Antihydrogen is made by mixing antiparticles called antiprotons (the antimatter counterparts of protons) and positrons (the antimatter counterparts of electrons). In recent months, Fujiwara said, the ALPHA team has figured out how to create very cold antiparticles and mix them more gently to produce and trap antiatoms more efficiently, successfully trapping an average of one antiatom per trial.
They also decided to test how long they could keep the antiatoms trapped. Fujiwara thought holding on to them for several seconds might be possible and was stunned that they could survive for many minutes.
That will make it much easier to do experiments to compare antihydrogen to hydrogen, he added.
For example, researchers can point lasers and microwaves at the antiatoms and figure out how the colours of light they shine back compare to those shone back by hydrogen atoms under the same circumstances, Fujiwara said.
Aiming the lasers and microwaves precisely at the antiatoms using the right settings is very difficult right after the antiatoms are formed because they are in what's called an excited state — the positrons are orbiting the nucleus of the antiatom, but they're very far away, and they're constantly changing their orbit.
Over time, they reach a stable orbit close to the nucleus known as the "ground state." That allows the lasers and microwaves to be aimed with high precision. Theoretical calculations show that after several minutes, the antiatoms should all be in the ground state.
Studying the effect of gravity on antimatter is particularly challenging because gravity acts so weakly compared to other forces on particles with such a tiny mass, so a lot of time is needed.
"The possibility of studying gravitational effects really become feasible now," Fujiwara said. "That’s something I’m personally interested in pursuing."
About one-third of the 40 physicists who make up the ALPHA collaboration are Canadian. The rest are from Brazil, Denmark, Israel, Japan, Sweden, the U.K. and the U.S.
Canadian funding for the project comes from NSERC (Natural Sciences and Engineering Research Council, TRIUMF, AIF (Alberta Ingenuity Fund), the Killam Trust, and FQRNT (Le Fonds québécois de la recherche sur la nature et les technologies).